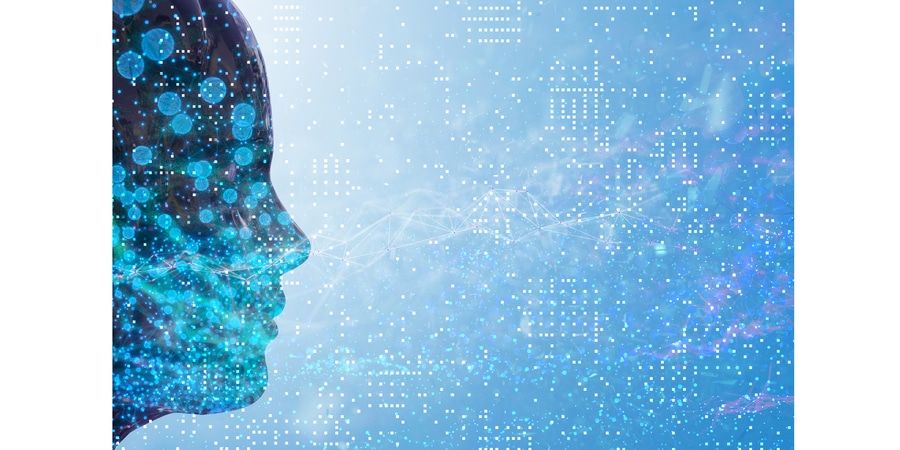
#Industry News
Series on transcranial Photobiomodulation Therapy
How it works in your brain
This article was originally published on the substack "Light and Equanimity", a free source of information about photobiomodulation therapy. You can subscribe to a more or less monthly newsletter:
https://lightandequanimity.substack.com/
Bottom Line
The main takeaways from this post are that transcranial PBM can in many cases help our brains restore or improve crucial natural processes at the core of neurological and mental health. Its most important effects are
- a higher ATP output and consequently an improved overall metabolism,
- improved cerebral blood and lymphatic flow,
- improved oxygenation,
- reduction neuroinflammation,
- antioxidant and antiapoptotic effects,
- improved neuronal signaling,
- improved neurogenesis,
- and improved synaptogenesis.
All these effects can help treat different neurological and psychological disorders.
Introduction
Among all the different applications of light in medicine, the field of transcranial Photobiomodulation therapy (tPBM) is my favorite one. The term describes the application of infrared light to the brain, which unfolds different beneficial effects.
What I like about it is, on the one hand, that we are talking about a natural therapy that is, if applied correctly, completely free of side effects. On the other hand, it allows therapists to treat disorders as a whole system instead of only acting on specific symptoms, which is difficult to achieve through conventional pharmacological interventions.
This post is an exploration of our current understanding of the mechanisms of action. Many mechanisms are complex and involve signaling cascades, secondary, and even tertiary effects, and are admittedly yet to be fully understood concerning all details.
However, we know that it works in what we can call a very natural way by enhancing and restoring different biological processes that lie at the core of good brain health. The therapy´s indications include
- neurodegenerative diseases,
- psychological disorders,
- brain injuries,
- headaches,
- improved cognitive function in healthy individuals,
- and neurodevelopmental disorders such as Autism.
How are tPBM-interventions executed?
“Near-infrared light” - defined as light of wavelengths between approx. 800nm and 1200nm – is applied directly on the head and penetrates through the skin, cranium, and all other tissues to eventually reach the brain. The ability of infrared photons to reach the brain tissue has been shown in different simulations and studies [1,2]. The deepest penetrating photons can reach a depth of up to 5cm, but the number of photos decreases with the distance traveled due to the absorption by the different biological tissues.
Specific wavelengths such as 810nm and 1024nm have been tested much more frequently than others due to the availability of particular products that emit these wavelengths.
These products can consist of either lasers or LEDs to emit the light. However, LEDs are much more common as they are significantly cheaper to produce and install in therapeutic devices.
The therapy is non-invasive, non-thermal, and painless.
Which mechanisms of action are at play?
More overall energy by increased Adenosine Triphosphate production
Adenosine Triphosphate (ATP) is the energy source used on a cellular level and thus often called the cellular “energy currency”. It is consumed in almost all basic physiological processes, including intracellular signaling, DNA and RNA synthesis, purinergic signaling, synaptic signaling, active transport, and muscle contraction.
A quick reminder: Its synthesis occurs within our mitochondria – an organelle in the cytoplasm of our human and almost all other eukaryotic cells.
There are three steps called
a) glycolysis,
b) tricarboxylic acid cycle (TCA or Krebs cycle),
c) oxidative phosphorylation.
T-PBM acts on the last step, oxidative phosphorylation, where ATP (as well as nitric oxide and reactive oxygen species) is produced through electron transfer processes in four different complexes [3].
The last complex is an enzyme called Cytochrome C Oxydase (CCO). There, copper (metal ions) reduces oxygen to water and ATP is produced in the process. What is essential in our context is that CCO can absorb red and infrared light photons.
The absorbed energy accelerates the electron transfer processes, making more electrons available in CCO, consequently leading to an increase in ATP output that directly follows the intervention [4,5].
Besides the immediate increase in ATP output, there are also long-term effects: When mitochondria sense that more energy is available, they signal this information to the nucleus. This alters the gene expression to enhance mitochondrial function and to form new mitochondria. The process is called retrograde mitochondrial signaling [6].
Keeping in mind that the brain is the organ with the highest energy consumption and has the second highest mitochondria density of all our organs (after the heart), it is particularly vulnerable to disruptions of its energy sources. Most neurodegenerative diseases (NDAs) are characterized by mitochondrial dysfunction, a loss of mitochondrial membrane potential, and depletion of ATP. Interventions that can improve ATP production and thus improve brain metabolism – such as tPBM – can therefore play a big part in a comprehensive strategy for improving cognitive function and possibly slowing or preventing cognitive decline [16].
Increased cerebral vasodilation
tPBM can improve cerebral blood flow and lymphatic flow by acting on nitric oxide in two different ways. First, a photodissociation of nitric oxide previously bound to the complex occurs during the photon absorption in Cytochrome C Oxydase. Secondly, tPBM can stimulate nitric oxide synthase, the enzyme necessary for NO production in tissues [7].
Nitric Oxide (NO) has vasodilatory effects. It thus leads to increased cerebral blood flow and cerebral lymphatic flow, which is crucial for supplying brain cells with nutrients and oxygen, removing waste products, and many other processes. Increased NO activity also leads to angiogenesis; the formation of new blood vessels.
A mouse model suggested that the effect size can be as big as 30% [8].
Improved cerebral oxygenation
Consistent with several animal studies, two human studies showed that transcranial laser stimulation can improve cerebral oxygenation in human adults [9, 10]. While total hemoglobin concentration only increases slightly to a non-significant portion, the oxygenated hemoglobin concentration increased and the deoxygenated hemoglobin concentration decreased, both significantly, leading to a significant increase in the share of oxygenated hemoglobin.
Decreased neuroinflammation
Neuroinflammation can contribute to the onset and progression of different neurological and psychological disorders, especially neurodegenerative diseases and depression, as they lead to neuronal loss and, thus, a decline in cognitive function.
TPBM can help decrease neuroinflammation in different ways. The mechanism can be simple - namely an improved removal of sources of inflammation such as metabolic waste products, neurotoxins, and pathogens. Through secondary effects, tPBM can also suppress the expression of proinflammatory cytokines [10]. In addition, a rodent model suggested that tPBM may also work via modulation of the brain´s immune system. Microglia are an essential part of it and exist in two main phenotypes. “Microglia 1” are responsible for producing proinflammatory cytokines and interrupting the blood-brain barrier and are, in general, associated with neuronal damage. “Microglia 2” produce anti-inflammatory cytokines, enhance neurotrophic factor release, and have neuroprotective effects. The rodent model showed that tPBM could shift the balance of the prevalent microglia phenotype from M1 to M2 [11].
Anti-oxidant effects
Long-term oxidative stress can contribute to neuronal loss, the disruption of neurocircuitry, a weakening of hippocampal, amygdalar, and cortical connections and can consequently lead to cognitive decline.
Due to the photon absorption in CCO, a brief and local burst of Reactive Oxygen Species (ROS) occurs. It leads to the activation of anti-oxidant pathways in the body. This reduces oxidative stress in the long run, despite ROS’s temporary and local increase [12].
Improved neurogenesis and synaptogenesis
Impaired neurogenesis is part of the neurobiology of different brain-related disorders and its correlation with cognitive impairment has been shown in different studies [13]. It seems to play a particularly important role in neurodegenerative diseases such as Alzheimer´s / dementia, but also in Autism.
tPBM can improve the processes that underly neurogenesis and synaptogenesis. The upregulation of the “Brain-Derived Neurotrophic Factor (BDNF)” - a signaling peptide involved in the maintenance and genesis of neurons and synapses - is the best-understood mechanism behind this effect.
Activation of signaling pathways and transcription factors that cause long-lasting changes in protein expression
Furthermore, tPBM activates multiple pathways through which signaling cascades occur, resulting in a long-term protein expression change.
The three best-understood pathways are:
a) The “calcium ions (CA²+) path”. The increased ATP production leads to a higher extracellular ATP level, which then leads to more Calcium influx into cells. The increased intra-cellular Calcium levels activate protein kinase C (PKC) and extracellular signal-regulated kinase (ERK) pathways or PI3K/Akt through calmodulin (CaM) [14]. These pathways play a crucial role in multiple cellular processes such as cell proliferation, differentiation, adhesion, migration, and survival.
b) Cyclic adenosine monophosphate (cAMP): Adenyl cyclase converts the more readily available ATP to the second messenger cAMP, which then activates PKA and Ras, which further leads to extracellular signal-regulated kinase and SIRT1 (Sirtuin 1) signaling [14]. The family of sirtuins has lately become pretty famous in integrative medicine and especially anti-aging medicine, mainly thanks to the work of Dr. David Sinclair, who was voted among the top 50 professionals in health care in 2018 and even one of the 100 most influential people in the world in 2014. In short, Sirtuins are a family of signaling proteins involved in metabolic regulation. Amongst other processes, SIRT 1 participates in neuronal plasticity, cognitive function, and mitochondrial biogenesis [15].
c) Reactive Oxygen Species (ROS): The aforementioned brief burst of ROS after the photon absorption in CCO leads to the activation of pathways like NRF2, and NF-kB. The NF-kB pathway (nuclear factor kappa light chain enhancer of activated B cells) is a family of highly conserved transcription factors regulating many important cellular behaviours, particularly inflammatory responses, cellular growth, and apoptosis [14].
Sources to obtain more information
Simply make contact with Martin Junggebauer to obtain more information about all the above: martin.junggebauer@gmail.com
All German-speaking readers can watch a webinar about the topic:
https://www.youtube.com/watch?v=ewFwWPs14Jw
Literature
[1] Salehpour F, Cassano P, Rouhi N, Hamblin MR, De Taboada L, Farajdokht F, Mahmoudi J. Penetration Profiles of Visible and Near-Infrared Lasers and Light-Emitting Diode Light Through the Head Tissues in Animal and Human Species: A Review of Literature. Photobiomodul Photomed Laser Surg. 2019 Oct;37(10):581-595. doi: 10.1089/photob.2019.4676. Epub 2019 Sep 25. PMID: 31553265.
[2] Li, Ting & Xue, Chang & Wang, Pengbo & Li, Yan & Wu, Lanhui. (2017). Photon Penetration depth in human brain for light stimulation and treatment: A Realistic Monte Carlo Simulation Study. Journal of Innovative Optical Health Sciences. 10. 10.1142/S1793545817430027.
[3] Bonora, M., Patergnani, S., Rimessi, A., De Marchi, E., Suski, J. M., Bononi, A., Giorgi, C., Marchi, S., Missiroli, S., Poletti, F., Wieckowski, M. R., & Pinton, P. (2012). ATP synthesis and storage. Purinergic signalling, 8(3), 343–357. https://doi.org/10.1007/s11302-012-9305-8
[4] Wong-Riley MT, Liang HL, Eells JT, Chance B, Henry MM, Buchmann E, et al. Photobiomodulation directly benefits primary neurons functionally inactivated by toxins: role of cytochrome C oxidase. J Biol Chem. (2005) 280:4761–71. doi: 10.1074/jbc.M409650200
[5] Karu T, Pyatibrat L, Kalendo G. Irradiation with He-Ne laser increases ATP level in cells cultivated in vitro. Photochem Photobiol B Biol. (1995) 27:219–23. doi: 10.1016/1011-1344(94)07078-3
[6] Dewey, C. W., Brunke, M. W., & Sakovitch, K. (2022). Transcranial photobiomodulation (laser) therapy for cognitive impairment: A review of molecular mechanisms and potential application to canine cognitive dysfunction (CCD). Open veterinary journal, 12(2), 256–263. https://doi.org/10.5455/OVJ.2022.v12.i2.14
[7] Chen, C., Hung, H. and Hsu, S. 2008. Low energy laser irradiation increases endothelial cell proliferation, migration and eNOS gene expression possibly via P13K signal pathway. Lasers Surg. Med. 40, 46–54
[8] Uozumi, Y., Nawashiro, H., Sato, S., Kawauchi, S., Shima, K. and Kikuchi, M. 2010. Targeted increase in cerebral blood flow by transcranial near-infrared irradiation. Lasers Surg. Med. 42, 566–576.
[9] Tian, F., Hase, S. N., Gonzalez-Lima, F., & Liu, H. (2016). Transcranial laser stimulation improves human cerebral oxygenation. Lasers in surgery and medicine, 48(4), 343–349. https://doi.org/10.1002/lsm.22471
[10] Holmes, E., Barrett, D. W., Saucedo, C. L., O'Connor, P., Liu, H., & Gonzalez-Lima, F. (2019). Cognitive Enhancement by Transcranial Photobiomodulation Is Associated With Cerebrovascular Oxygenation of the Prefrontal Cortex. Frontiers in neuroscience, 13, 1129. https://doi.org/10.3389/fnins.2019.01129
[11] Tang, Y. and Le, W. 2016. Differential roles of M1 and M2 microglia in neurodegenerative diseases. Mol. Neurobiol. 53, 1181–1194.
[12] Bathini, M., Raghushaker, C.R. & Mahato, K.K. The Molecular Mechanisms of Action of Photobiomodulation Against Neurodegenerative Diseases: A Systematic Review. Cell Mol Neurobiol 42, 955–971 (2022). https://doi.org/10.1007/s10571-020-01016-9
[13] Li Puma, D. D., Piacentini, R., & Grassi, C. (2021). Does Impairment of Adult Neurogenesis Contribute to Pathophysiology of Alzheimer's Disease? A Still Open Question. Frontiers in molecular neuroscience, 13, 578211. https://doi.org/10.3389/fnmol.2020.57821
[14] Caldieraro, M. A., & Cassano, P. (2019). Transcranial and systemic photobiomodulation for major depressive disorder: A systematic review of efficacy, tolerability and biological mechanisms. Journal of affective disorders, 243, 262–273. https://doi.org/10.1016/j.jad.2018.09.048
[15] https://www.sciencedirect.com/topics/neuroscience/sirtuin-1