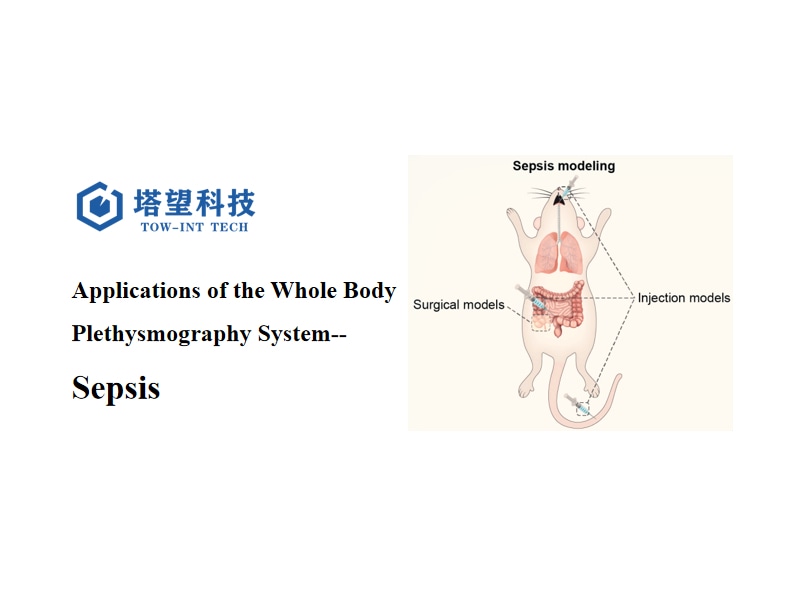
#Industry News
Applications of the Whole Body Plethysmograph in Sepsis
Sepsis can lead to ARDS, and the WBP as an important pulmonary function testing tool, is often used in animal experiments.
1. Background Introduction of Sepsis
Sepsis is a severe systemic reaction triggered by a bacterial infection, but it can also be caused by infections from viruses, fungi, or other pathogens. It can lead to organ dysfunction, insufficient tissue perfusion, and abnormal cellular metabolism. When the body's response to an infection gets out of control and starts to damage its own tissues and organs, sepsis occurs. It can rapidly progress to severe sepsis with acute organ dysfunction and even develop into septic shock, which is characterized by a significant drop in blood pressure, and the inability to maintain sufficient blood flow to vital organs even with fluid resuscitation. Common sources of infection include pulmonary infections (such as pneumonia), urinary tract infections, intra-abdominal infections (such as a perforated appendix), skin infections, etc. Any type of infection has the potential to trigger sepsis under suitable conditions.
The Whole Body Plethysmography (WBP) system can be used to monitor the respiratory function of experimental animals that are awake, freely moving, unbound, and not anesthetized, as well as to detect airway hyperresponsiveness. It avoids the effects of traumatic tracheotomy and anesthesia. The animals can survive after the experiment, making it an ideal experimental method for long-term follow-up studies. It can test multiple animals simultaneously, making it the best choice for screening experiments.
The WBP system can be used in sepsis research and is also particularly suitable for high-throughput studies such as drug primary screening, pharmacological and efficacy analysis, and toxicological analysis, as well as long-term follow-up studies. The respiratory parameters measured by this system, including respiratory rate, tidal volume, minute ventilation volume, peak expiratory flow, peak inspiratory flow, expiratory time, inspiratory time, and the ratio of peak expiratory flow time (Rpef), have a high correlation with the results of classical pulmonary function tests.
2. Commonly Used Mouse Sepsis Models
2.1 Cecal Ligation and Perforation (CLP) Model:
This is one of the most commonly used animal models of sepsis. It induces intraperitoneal infection by surgically partially ligating and perforating the cecum, thus simulating sepsis caused by intra-abdominal endogenous infection in humans. The CLP model is considered to be close to the clinical situation of sepsis because it involves a complex mixture of pathogens and the progressive development of multiple organ dysfunction.
2.2 Lipopolysaccharide (LPS) Injection Model:
LPS is the main component of the outer membrane of the cell wall of Gram-negative bacteria. Injecting LPS intravenously or intraperitoneally can induce a systemic inflammatory response similar to that of human sepsis. Although this model is easy to operate, it mainly causes an acute inflammatory response and lacks the typical multi-stage characteristics of sepsis.
2.3 Bacterial Inoculation Model:
Inject specific types of live bacteria (such as Escherichia coli, Staphylococcus aureus, etc.) directly into the animal's body to induce local or systemic infection, which then develops into sepsis. This method allows for the adjustment of the severity of the disease by selecting different types of bacteria and doses as needed.
2.4 Intratracheal Instillation Model:
Intratracheal instillation of bacteria or their products (such as LPS) is used to simulate sepsis caused by pulmonary infection. This method is particularly suitable for studying sepsis related to pneumonia.
3. The Model ofAcute Respiratory Distress Syndrome(ARDS)Associated with Sepsis in Mice is Induced by the Method of Cecal Ligation and Perforation (CLP). The Specific Steps are as Follows:
Preparation of Experimental Animals: Select healthy male C57BL/6 mice aged 6-8 weeks and weighing 22 ± 2g.
Anesthesia Treatment: Anesthetize the mice with isoflurane.
Surgical Operation: After the anesthesia takes effect, expose the cecum of the mouse. Ligate the cecum about 1 cm from the end of the cecum. Then, puncture the cecum with a 16-gauge needle at a position 0.5 cm from the end of the cecum. After completing the above operations, suture the incision layer by layer.
Postoperative Resuscitation: After the operation, help the mice recover by subcutaneously injecting 0.5 ml of pre-warmed sterile normal saline on the back of the mice.
Setting up the Control Group: For the control group mice, the cecum is not ligated or perforated, and the remaining surgical procedures are the same as those of the model group.
Drug Administration: Immediately after the model establishment, the mice in the GL + CLP group are intraperitoneally injected with glycyrrhizic acid (GL, 15mg/kg). GL is dissolved in 200 μl of the solvent (2% DMSO + 40% peg300 + 5% Tween80 + 53% ddh2O).
Pulmonary Function Detection: Perform pulmonary function detection on the model group and the blank group mice 16-20 hours after the operation.
Specimen Collection: Obtain the required specimens 24 hours after the operation.
4. Evaluation Methods of Sepsis
4.1 Observe the 7-day Survival Rate:
Evaluate by observing and recording the survival status of the mice within 7 days. The survival rate of the mice in the CLP group is low. 80% of them survive at 24 hours, and only 10% survive at 7 days, and there are no more deaths after 72 hours. While the 24-hour survival rate of the GL+CLP group is 85%, and the 7-day survival rate is 40%, and there are no deaths after 42 hours. The survival rate is significantly lower than the normal level, which is in line with the characteristics of severe sepsis and high fatality rate, and indirectly reflects the successful establishment of the model.
4.2 Pathological Examination of Lung Tissue:
Perform hematoxylin-eosin (HE) staining on the mouse lung tissue, observe the pathological changes of the lung tissue, and calculate the lung injury score. Compared with the control group, the lung tissue of the mice in the CLP group is severely damaged. The alveolar structure is destroyed, the pulmonary interstitium is significantly thickened, accompanied by bleeding and a large number of inflammatory cell infiltrations, and the lung injury score is significantly increased. These pathological features are consistent with ARDS induced by sepsis, indicating the successful establishment of the model.
4.3 Detection of the Wet/Dry Ratio (W/D) of Lung Tissue and the Protein Concentration in Bronchoalveolar Lavage Fluid (BALF):
The W/D ratio of the lung and the protein concentration in BALF of the mice in the CLP group increase significantly. These two indicators are important indicators reflecting the damage of the alveolar-capillary barrier and the increased permeability of the lung tissue during ARDS. The changes in these indicators indicate that the model mice have pathological changes similar to ARDS, supporting the successful establishment of the sepsis model.
4.4 Detection of the Formation of Neutrophil Extracellular Traps (NETs) in Lung Tissue:
Detect the content of Cit-H3 (a marker of NETs) in the lung tissue by immunofluorescence staining. The content of Cit-H3 in the lung tissue of the mice in the CLP group is higher than that in the control group, which means that the formation of NETs increases. Since NETs are related to the progression of sepsis, the increase in their amount indicates that a sepsis-related immune response has occurred in the mice, which is one of the evidences for the successful establishment of the model.
4.5 Detection of the Expression of Proteins and Genes in the Related Signaling Pathways:
Use western blot and quantitative real-time PCR to detect the protein and gene expression levels of HMGB1, TLR9, MyD88, and IL6 in the lung tissue respectively. The expression of these molecules in the CLP group increases significantly. They are involved in the regulation of the inflammatory response and the formation of NETs in sepsis, and the changes in their expression are consistent with the pathological mechanism of sepsis, further confirming the successful establishment of the model.
4.6 Evaluation of Pulmonary Function:
The pulmonary function of the mice can be detected using the Whole Body Plethysmography system developed by TaWang Technology. Many pulmonary function indicators of the mice in the CLP group change significantly compared with those in the control group. For example, F, Vt, and MV, which reflect alveolar ventilation, decrease, indicating a reduction in alveolar ventilation; PIF, PEF, and Rpef, which reflect the strength of the respiratory muscles and small airway obstruction, decrease, indicating the occurrence of respiratory fatigue and airflow limitation; Ti and Te are prolonged, indicating the presence of respiratory obstruction or pulmonary edema; EF50 increases and Tr is prolonged, indicating an increase in airway resistance. These changes in pulmonary function are consistent with the characteristics of ARDS induced by sepsis, indicating the successful establishment of the model.
5. Tow-Int Tech - Whole Body Plethysmography System, WBP
The Whole Body Plethysmography (WBP) system developed by Tow-Int Tech can measure the respiratory parameters of awake and freely moving animals, such as respiratory rate, tidal volume, and Airway Hyperresponsiveness (AHR) testing. During the test, the animals can be in a state of wakefulness and freedom, avoiding the effects of traumatic tracheotomy and anesthesia, making the experimental process more convenient. It is used for the study of the responsiveness of animals in respiratory system models to drugs and other substances, as well as the pharmacological and toxicological studies of respiratory drugs. It is particularly suitable for rapid primary screening tests of a large number of animals, long-term follow-up studies, and repetitive screening.
Applicable Animal Species: Mice, young mice, rats, guinea pigs, rabbits, dogs, cats, miniature pigs, monkeys, and other animals.
It is equipped with a feeding and watering device to facilitate ultra-long-term experiments.
Measurement Channels: 1-64 channels.
Automatic bias flow control function.
It can be configured with a high-frequency oscillating nebulization drug delivery system.
It has a special noise reduction structure design, which can effectively reduce the interference caused by environmental changes.
It can perform bias flow meter noise reduction to improve the signal-to-noise ratio of the signal and reduce system noise.
It supports the external connection of nitrogen or other gases to complete hypoxic experiments.
It has analysis software, and the data can be saved in excel or txt format.
The software can automatically switch up to four channels of different gases and can be operated through an external controller.
References
[1]Gu J, Ran X, Deng J, et al. Glycyrrhizin alleviates sepsis-induced acute respiratory distress syndrome via suppressing of HMGB1/TLR9 pathways and neutrophils extracellular traps formation[J]. International immunopharmacology, 2022, 108: 108730.
[2]Ruan D, Yang J, Luo Q, et al. The protective effects of Goitrin on LPS-induced septic shock in C57BL/6J mice via Caspase-11 non-canonical Inflammasome inhibition[J]. Molecules, 2023, 28(7): 2883.
[3]Silva-Santos Y, Pagni R L, Gamon T H M, et al. Lisinopril increases lung ACE2 levels and SARS-CoV-2 viral load and decreases inflammation but not disease severity in experimental COVID-19[J]. Frontiers in Pharmacology, 2024, 15: 1414406.
[4]Sordillo P P, Allaire A, Bouchard A, et al. The complex lipid, SPPCT-800, reduces lung damage, improves pulmonary function and decreases pro-inflammatory cytokines in the murine LPS-induced acute respiratory distress syndrome (ARDS) model[J]. Pharmaceutical Biology, 2022, 60(1): 1255-1263.
[5]Li H, Zou Q, Wang X. Bisdemethoxycurcumin alleviates LPS-induced acute lung injury via activating AMPKα pathway[J]. BMC Pharmacology and Toxicology, 2023, 24(1): 63.
[6]Rivera J, Nakouzi A, Abboud N, et al. A monoclonal antibody to Bacillus anthracis protective antigen defines a neutralizing epitope in domain 1[J]. Infection and immunity, 2006, 74(7): 4149-4156.
[7]Domachowske J B, Bonville C A, Rosenberg H F. Animal models for studying respiratory syncytial virus infection and its long term effects on lung function[J]. The Pediatric infectious disease journal, 2004, 23(11): S228-S234.
[8]de Jong R, Nuiten W, Ter Heide A, et al. Adapting Real-Time Lung Function Measurements for SARS-CoV-2 Infection Studies in Syrian Hamsters[J]. Viruses, 2024, 16(7): 1022.
Contact us now!
We are committed to making your research easier, more accurate, and more efficient and helping you build confidence in your data! We have provided services for a large number of customers and have rich experiences in offering customized, professional solutions according to your needs.