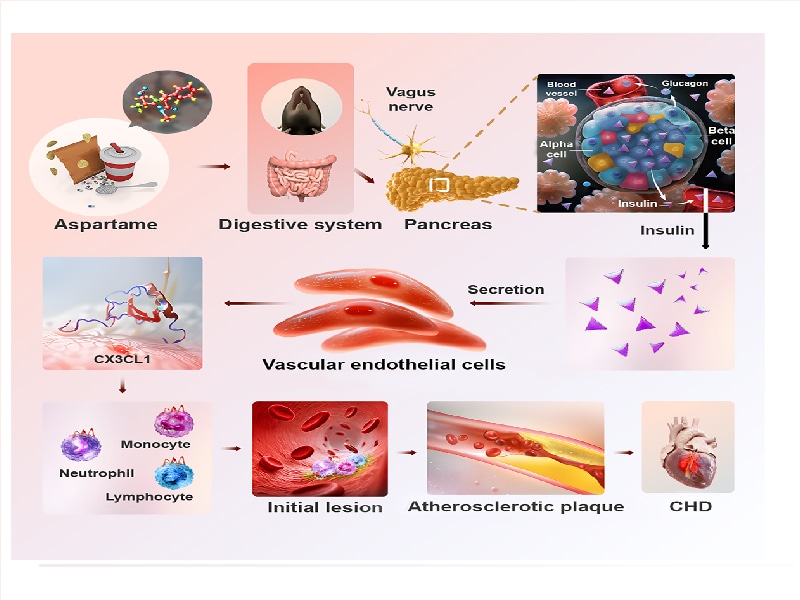
#Industry News
Aspartame: A Sweet Trap or a Health Minefield?
Research shows aspartame raises insulin via vagus nerve, worsens atherosclerosis, and Cx3cr1 knockout is a new therapy target.
Recently, the research team led by Academician Cao Yihai from Karolinska Institute in Sweden collaborated with the teams led by Academicians Zhang Yun, Zhang Cheng, and Dean Chen Yuguo from Qilu Hospital of Shandong University. They published a research paper titled "Sweetener aspartame aggravates atherosclerosis through insulin-triggered inflammation" in the journal Cell Metabolism. This study points out that aspartame can exacerbate the occurrence of atherosclerosis and increase the risk of cardiovascular diseases. Although artificial sweeteners (such as aspartame) can limit sugar intake and reduce energy storage, why do they cause cardiovascular diseases related to fat accumulation?
Research Background
In July 2023, the World Health Organization classified aspartame, one of the most common artificial sweeteners, as a Group 2B carcinogen, which once triggered widespread concern about artificial sweeteners from all sectors. Of course, Group 2B only means that it is possibly carcinogenic to humans, and the evidence of its carcinogenicity to humans is limited, so there is no need to panic excessively. However, apart from cancer, the associations between artificial sweeteners and other diseases are constantly emerging.
Research Objectives
This study aims to explore the association between artificial sweeteners (ASWs), especially aspartame (APM), and cardiovascular diseases (CVDs). It focuses on analyzing the specific molecular mechanisms by which APM affects atherosclerosis, providing a theoretical basis and potential therapeutic targets for the prevention and treatment of cardiovascular diseases.
Figure 1: Specific molecular mechanisms by which APM affects atherosclerosis
Experimental Methods
Mouse models: ApoE-/- mice and Cx3cr1 MΦ−/−/ApoE −/− double knockout mice, all fed with a high-fat and high-cholesterol diet (HFCD)
Cynomolgus monkey model: Four-year-old male cynomolgus monkeys were used. APM or sucrose was added to their drinking water to simulate the scenario of human intake of sweeteners. The changes in indicators such as insulin, glucose, and C-peptide in the blood were detected to verify whether the effect of APM on insulin secretion found in the mouse model also exists in primates, enhancing the reliability and universality of the research results.
Technical means
Gene editing technology: Technologies such as CRISPR-Cas9 were used to construct gene-modified mouse models. For example, LoxP sites were inserted on both sides of the Cx3cr1 gene and then crossed with Lyz2-Cre mice to knockout the Cx3cr1 gene in monocytes/macrophages and study its function.
Molecular biology techniques: RNA sequencing was used to analyze the gene expression profile of insulin-stimulated PAECs to find potential signaling molecules; qPCR was used to measure the mRNA levels of specific genes (such as Cx3cl1); Western blot was used to detect the expression of related proteins (such as CX3CL1 and CX3CR1) to explore the change patterns of related molecules when APM affects atherosclerosis.
Cell biology techniques: The primary cell culture technique was used to isolate and culture mouse PAECs and primary macrophages for in vitro studies of cell-cell interactions and signal transduction. Through parallel plate flow chamber experiments and Boyden chambers experiments, the adhesion between monocytes and endothelial cells and the migration ability of monocytes were studied to explore the effect of the CX3CL1-CX3CR1 signaling pathway on cell functions.
Metabolic detection techniques: The animal energy metabolism detection system was used to measure the respiratory metabolism parameters of mice, including oxygen consumption, carbon dioxide production, heat production, etc., to evaluate the overall metabolic effect of APM on mice; the glucose tolerance test (GTT) and insulin tolerance test (ITT) were used to detect the insulin sensitivity of mice to determine whether APM leads to insulin resistance.
Single-cell sequencing: It was used to analyze the population characteristics and gene expression differences of peripheral blood monocytes (PBMCs) in Cx3cr1 lox/lox and Cx3cr1 MΦ−/− mice, to gain an in-depth understanding of the changes and mechanisms of action of different cell populations during the process of APM affecting atherosclerosis.
Experimental Results
1. APM exacerbates atherosclerosis in mice:
After feeding ApoE-/- mice with different doses of APM for 12 weeks, the atherosclerotic plaques (APs) in the aorta of the APM-fed group increased significantly and showed a dose-dependent relationship. The time-course experiment showed that when the mice were fed with APM for 4 weeks, the number of atherosclerotic lesions and the average plaque size increased, and they further increased at 8 weeks and 12 weeks. The control group fed with 15% sucrose showed no obvious development of APs at 4 weeks and 8 weeks, and the promoting effect only appeared at 12 weeks. Feeding with APM did not affect the body weight, fat mass composition, food intake, and most metabolic parameters of the mice. Only feeding with sucrose slightly increased the body weight and fat mass composition of the mice, and the serum LDL decreased slightly.
Figure 2: APM exacerbates atherosclerosis in mice
2. APM increases plasma insulin levels and regulates insulin through the vagus nerve:mice:
After feeding mice with different concentrations of APM, the plasma insulin levels increased significantly within 30 minutes. Continuous feeding with 0.15% APM for 12 weeks led to a continuous increase in serum insulin levels, and the mice developed insulin resistance. The cynomolgus monkey experiment also showed that after feeding with 0.15% APM or 15% sucrose for 10 minutes, the insulin levels increased transiently at 30 minutes and returned to normal at 60 minutes, and the insulin levels in the APM group and the sucrose group were similar, but the changes in blood glucose levels were opposite. Bilateral subdiaphragmatic vagotomy (SDV) could completely inhibit the insulin production and atherosclerosis induced by APM.
Figure 3: APM increases plasma insulin levels and regulates insulin through the vagus nerve
3. Mechanistic study of insulin-dependent atherosclerosis:
Implanting an insulin-releasing micropump into ApoE-/- mice to simulate the increase in circulating insulin levels in humans. After 4 weeks, the formation, growth, and instability of APs were exacerbated, which was similar to the effect of APM feeding. Using streptozotocin (STZ) to eliminate pancreatic insulin production could completely counteract the enhanced formation, growth, and instability of APs caused by APM, demonstrating that the upregulation of insulin is a necessary condition for APM to exacerbate atherosclerosis.
Figure 4: Mechanistic study of insulin-dependent atherosclerosis
4. CX3CL1 is an insulin-stimulated arterial endothelial chemokine:
After insulin stimulated primary aortic endothelial cells (PAECs) for 8 hours, RNA-seq analysis showed that Cx3cl1 was the most significantly upregulated gene, and qPCR and Western blot verified the high expression of its mRNA and protein. In vivo experiments showed that the levels of both circulating and vascular endothelial cell membrane-bound CX3CL1 in APM-fed mice increased significantly, and this increase was dependent on insulin.
Figure 5: CX3CL1 is an insulin-stimulated arterial endothelial chemokine
5. Chemotactic and adhesive effects of CX3CL1-CX3CR1 on monocytes/macrophages:
In RAW264.7 monocytes, the CX3CR1 protein was highly expressed. Knocking down CX3CR1 with shRNA could inhibit the adhesion of insulin-enhanced monocytes to PAECs. The parallel plate flow chamber experiment showed that insulin stimulation of PAECs could increase the adhesion of monocytes, and knocking down CX3CR1 could inhibit this effect. In addition, the CX3CL1-CX3CR1 signaling pathway could also induce the migration of monocytes and the polarization of M0 macrophages into M1 macrophages.
Figure 6: Chemotactic and adhesive effects of CX3CL1-CX3CR1 on monocytes/macrophages
6. Deletion of the Cx3cr1 gene reduces the pro-inflammatory myeloid cell population:
Constructing Cx3cr1 MΦ−/− mice, single-cell RNA sequencing (scRNA-seq) analysis showed that the myeloid cell population was significantly reduced, the subpopulations of monocytes/macrophages changed, the Mono-c1 subpopulation decreased, and the Mono-c3 and Macro-c5 subpopulations increased. Pseudo-time analysis showed that the CX3CL1-CX3CR1 signaling axis affected monocyte differentiation.
Figure 7. scRNA-seq of PBMCs in Cx3cr1MΦ–/– mice
7. Loss of function of the Cx3cr1 gene in monocytes/macrophages counteracts the atherosclerosis exacerbated by APM:
Compared with the control group, the enhanced formation and growth of APs caused by APM were completely counteracted in Cx3cr1 MΦ−/−/ApoE −/− double knockout mice. In the early stage (4 weeks) of AP development, the deletion of the Cx3cr1 gene significantly inhibited lesion formation, and the anti-atherosclerotic effect was more obvious at 8 weeks and 12 weeks.
Figure 8. Genetic loss of function of Cx3cr1 in monocytes/macrophages neutralizes APM-exacerbated atherosclerosis
Research Conclusion
The study found that aspartame can increase insulin levels by activating the parasympathetic nerve, thereby exacerbating atherosclerosis, and this process is insulin-dependent and involves the CX3CL1-CX3CR1 signaling pathway.
Specifically knocking out the Cx3cr1 gene in monocytes/macrophages can counteract the atherosclerosis exacerbated by aspartame, providing a new target for the treatment of related cardiovascular diseases.
In this study, the animal energy metabolism system was used to monitor the oxygen consumption, carbon dioxide production, heat production and other indicators of mice, providing key data support for exploring the relationship between aspartame and atherosclerosis, indicating that energy metabolism monitoring plays an important role in life science research.
Our company, Tow-Int Tech, independently developed an animal energy metabolism detection system, which can be used for real-time monitoring and recording of metabolic and motor-related indicators of small animals, and can qualitatively and quantitatively measure and analyze the behavioral activities of animals and their interactions with respiratory metabolism. The detected indicators include oxygen consumption, CO2 production, RER respiratory exchange ratio, EE energy consumption, food intake, water intake, XYZ three-dimensional movement, body weight, running wheel activity and other indicators.
References:
[1] Wu W, Sui W, Chen S, et al. Sweetener aspartame aggravates atherosclerosis through insulin-triggered inflammation[J]. Cell Metabolism, 2025.
Contact us now!
We are committed to making your research easier, more accurate, and more efficient and helping you build confidence in your data! We have provided services for a large number of customers and have rich experiences in offering customized, professional solutions according to your needs.